What about the future? What are the new applications? And can we really use this to communicate with exoplanets?
How can operators benefit from digital beamforming? What is the capacity gain and why does it work so much better?
Drilling into the hart of the matter for BeammWave! Digital beamforming – to-be-or-not-to-be?
Everything goes digital, why would beamforming be the only technology that does not?
What is mmWave and how do you do beamforming?
The mmWave frequency range is highly suitable for sensing and radar purposes due to the short radio wavelength enabling localization and positioning in the mm to cm range. Joint sensing and communication is being discussed, where a communication system not only communicates but also senses the environment and basically uses mmWave radar for the detection and positioning of objects.
Traditionally when designing analog or hybrid beamforming solutions for mmWave the design has been targeting a single application. A certain antenna panel/array has been designed for a certain purpose, such as a handheld device, and if another mmWave transceiver architecture is needed for another application that needs more antennae, such as a fixed wireless access point, or a small base station, another larger antenna panel has typically needed to be designed.
The 5G-NR mmWave communication devices range from a simple IoT device, via smartphones and fixed wireless access points, to devices for non-terrestrial communication as well as base stations. Designing different antenna panels with analog beamforming solutions for each of these use cases comes with a cost.
It would be an advantage to develop a scalable beamforming solution covering several of the use cases above, thereby reducing the development cost and enabling innovation of potential not-yet-known applications (possibly having almost unlimited bandwidth) as is the case in the mmWave radio spectrum.
Fortunately a digital beamforming architecture where one integrates the radio transceiver (RF) chip and antenna in a single encapsulation has this scaling possibility. The radio chip comprises the analog front end radio components, including down and upconverters, taking the mmWave radio signal from mmWave radio frequency down to an analog baseband signal on the receiver side and converting the transmitted analog baseband signal to mmWave radio signals on the transmitter side. The analog baseband signals have a bandwidth corresponding to the 5G-NR mmWave bandwidth and therefore are in the range of 100-400 MHz, those signals being easy to route on the PCB to a digital baseband processor.
The above integrated antenna and RF chip, when combined with digital beamforming algorithms that are implemented in the digital baseband processor, can be tailored to a flexible amount of antennae. Thus a scalable, low cost mmWave implementation for various use cases can be achieved by just applying as many RF chips as are needed for the particular use case. For instance, if we consider a mmWave IoT device that is only transmitting a small amount of data over short ranges, then it may only need two RF chips, plus associated SW that handles beamforming, to be configured for connection to two antennae to create such a device.
A more complex use case relates to a mmWave smartphone implementation. This needs to solve the challenges associated with handheld devices (these have been discussed in previous posts) and therefore need a distributed antenna architecture with, say, 8-16 Antennas and RF chips, with the corresponding digital beamforming algorithms adapted to that amount of antennae. Considering even more complexity, such as a Fixed Wireless Access Point, then 32-64 RF chips may be needed so as to achieve the desired data rate (several Gb/s).
The same set of mmW RF chips are reused for all types of devices. However, in order to meet any requirements for higher transmitting power and better receiver sensitivity, typical for more advanced use cases, then these are solved by adding more RF chips. The level of scaling can also continue to base stations, devices used for non-terrestrial communications such as drones and aeroplanes (requiring 100+ antennae) and to devices communicating with satellites (1000+ antennae). One can even imagine extending the scaling idea of digital beamforming radio architectures to inter-planetary and interstellar communication, where the number of antennae in these cases need to be in the range of hundreds of thousands to hundreds of millions of antennae. However, there might be some challenges with the 5G latency requirements in the communication that need to be solved as well
BeammWave develops a scalable digital beamforming architecture enabling the mmWave mass market on earth as well as in the sky.
We have in our previous posts shown the advantages with digital beamforming over analog beamforming from a performance point of view. Then one may ask; why hasn’t digital beamforming in mobile devices happened yet?
Well, if you ask people in the field, they will say that a digital approach has way to high power consumption due to the need of entire transceiver chain for all antennas whereas an analog approach only requires single transceiver chain due to that the beamforming is done in the analog domain at the front-end receiver using phase-shifters. Well, this might have been the truth in the past but let us check the case with the current state of the art technology.
Starting with the analog phase-shifters, which comprises switches and various physical routing of traces on the PCB used in order to make sure of coherent combining of the signal received or transmitted from the respective antenna. Passive phase shifters come with a loss of 8-10 dB to the already weak radio signal received by the antenna. That signal power loss needs to be compensated by a high gain Low Noise Amplifiers (LNA) on the receiver side and a high gain Power Amplifier (PA) design on the transmitter side. Such high gain high LNAs and PAs consume a significant part of the total power consumption in the radio receiver.
A digital beamforming architecture performs the combining in the digital domain and therefore the LNA and PA design can be more relaxed for digital architectures saving significant amounts of the power consumption.
Another important factor is that it is commonly believed that the analog-to digital and digital-to analog converters (ADC,DAC) need to be designed in the same way for both digital and analog beamforming, and since a digital beamforming requires an ADC/DAC pair for each antenna while the analog one only requires a single pair of ADC/DAC the power consumption must be N times larger for these components if N antennas is used. Furthermore, ADC/DAC has also in the past been a very power-hungry component and that (today erroneous) fact is still in many people’s mind in the field.
In fact, the power consumption for ADC/DACs especially when using 4-8 bits resolution, which is what is sufficient from a handheld device point of view, is nowadays, with evolving chip technology, on par with other radio components. Furthermore, which might at a first glance be a bit surprising, is that the number of bits needed for digital beamforming architectures can be reduced with 1 – 2 bits compared to an analog beamforming implementation due to the inherent converter quantization noise suppression made by combining the receiver streams after the ADC instead of before the converters as in the analog case! This relaxes the power consumption for the ADCs in digital beamforming solutions with 50-75% compared to the ADC needed for the analog solution!
By understanding and utilizing these facts together with a careful digital beamforming system design, BeammWave can deliver a sustainable and scalable digital beamforming solution with power consumption on par or better than current analog beamforming solutions for mobile devices! For further information please check out our white paper on The Power Efficient Architecture for 5G on mmWave Frequencies.
Chips today for consumer electronics are practically all done using a technology known as CMOS (Complementary Metal Oxide Semiconductor, silica-based). However, for the high frequency parts in a radio transceiver which are present in all communication devices, another technology based on gallium compounds has been considered better suited in terms of high frequency performance. However, this technology has been limited by the fact that integration of different parts is more complicated and the production cost is relatively high. Therefore, in comparison, CMOS technology has several advantages as it is easy to integrate different circuit functions on the same chip and the manufacturing cost for large volumes is low per unit.
Wireless mmWave communication uses several different high frequency bands (28GHz, 39GHz, etc.). The traditional approach would therefore be to design several different chips covering the corresponding frequency bands. However, the combination of different frequency bands into one chip would have several advantages, such as minimising the number and length of connections and thereby internal losses. In addition, losses are also heavily reduced if we then integrate the chips with antennas in packages.
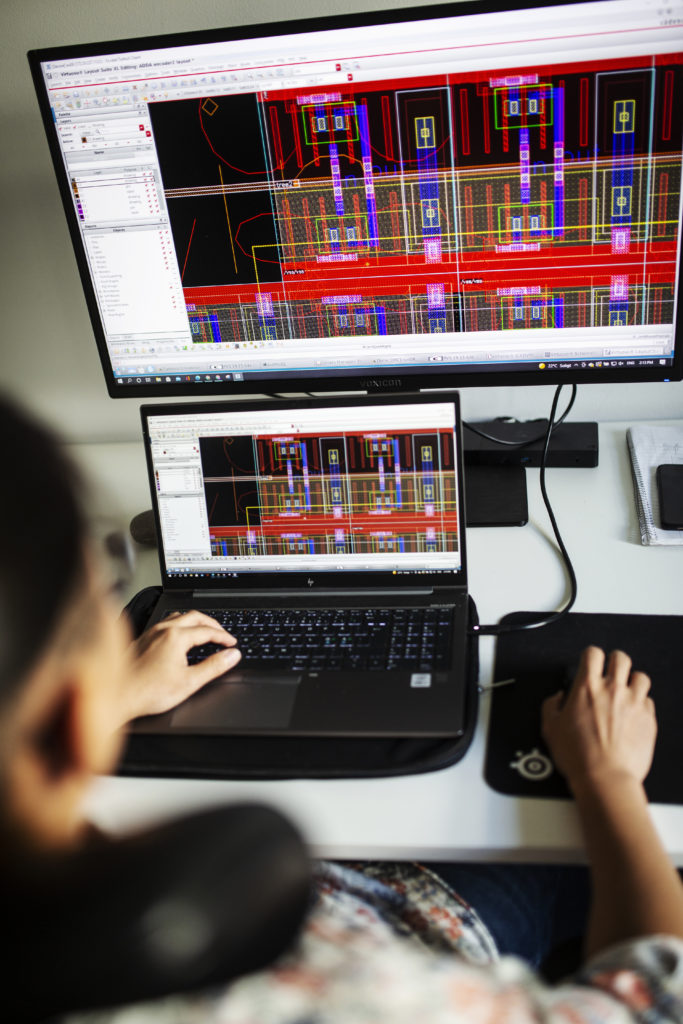
It can therefore be summarized that it’s very beneficial if a chip covers several frequency bands, integrates several circuit functions into one chip, is easy to manufacture and has a low production cost per unit. It is also advantageous if the chip is power efficient, especially if it will be used in battery powered devices.
BeammWave’s know-how of the system architecture, including digital beamforming algorithms, mmWave radio, and baseband interface allows us to optimize both the hardware and software for high system performance, i.e. we hunt down optimal performance in the complete system of which the mmWave radio is one part of. This concept allows us to comply with today’s stringent demands on radio design; a small physical size (without need for external power amplifiers), power efficiency, fast interface, and useful in different applications (smartphones, Consumer Premises Equipment (CPE), micro base stations, IoT devices). Finally, this approach gives us a more scalable and energy efficient digital beamforming solution for mmWave frequencies.
Innovation is the strangest mix of slow and lightning fast. The slow part is all the tedious research done in labs and at universities, the hard (and slow work) of specifications, and the macro trends building persistently. These are all necessary enablers, but are for the most time not recognized as groundbreaking inventions, even if they sometimes render a Nobel Prize decades after they were done.
The missing part, and what we often perceive as the innovation that moves at the speed of light, is when the application is added on top of the new technology. This allows the full power of the underlying technology to be productive and generate a benefit for its users. The real trick here is to get the timing right and, as always, understanding the economic drivers and prerequisites for success.
If we focus on the use of mmWave in 5G, all the fundamental research and specification work has been done and we now have a VERY potent technology at our disposal. 5G has a few bold promises with “endless” capacity, “no” latency and “fiber like” speed. This enables innovations like remote surgery, remote control of different types of vehicles, industrial automation, virtual presence, etc.
BUT none of the above applications will be drivers of 5G. They will (almost) all happen once mmWave is widely deployed and available, but for none of them, on an individual basis, will it make financial sense to take the full, or even part, of a network investment. This is nothing new since it seems like every amazing and promising technology is always looking for the “killer app”, the one application that on its own can justify the wide deployment of the base technology. Once this is done and the technology is deployed, there will be an avalanche of innovative use cases and devices being built. I believe that many of us have been in the situation where we have tried to orchestrate a technology liftoff by trying to use the collective power of many different applications and, unfortunately, I believe there are almost as many of us who have failed, myself included. It simply seems to be a universal truth that a single killer app is needed.
This is probably also the explanation to the phenomena that so many have observed over and over again, namely that promising technologies always impact the market later than expected but once it happens the impact is way bigger than anyone would have dreamed of. It takes something to start an avalanche, but once started there is nothing stopping it!
So, what is the killer app for mmWave and 5G?
I will say…the smartphone!
But wait a minute, that was the killer app already for 4G! Yes, that is true … and your expectation of that killer app is an increased data bucket at a lower price. The operator needs a constantly lower price per bit sent and that is not possible without new technology and new frequency bands like mmWave. I also know that BeammWave’s digital beamforming solution will be one of the key enablers for making mmWave in 5G happen for the mass market.
Many believe the main reason for introducing communication in the mmWave frequency range (24 GHz and above) in 5G-NR is to allow for use cases requiring Gb/s such as VR or AR use cases. It is true that such use cases require a lot of bandwidth not existing in current sub 6 GHz frequency bands, and it is also true that 5G will be an unprecedented innovation platform for the coming decade, however the main driver is the need for more capacity in the radio spectrum.
Data consumption explosion over cellular is expected to grow exponentially and hence there will be a time, predicted to be around 2023 starting in Mega cities, where communication over the sub 6 GHz frequency bands start to reach its capacity limits.
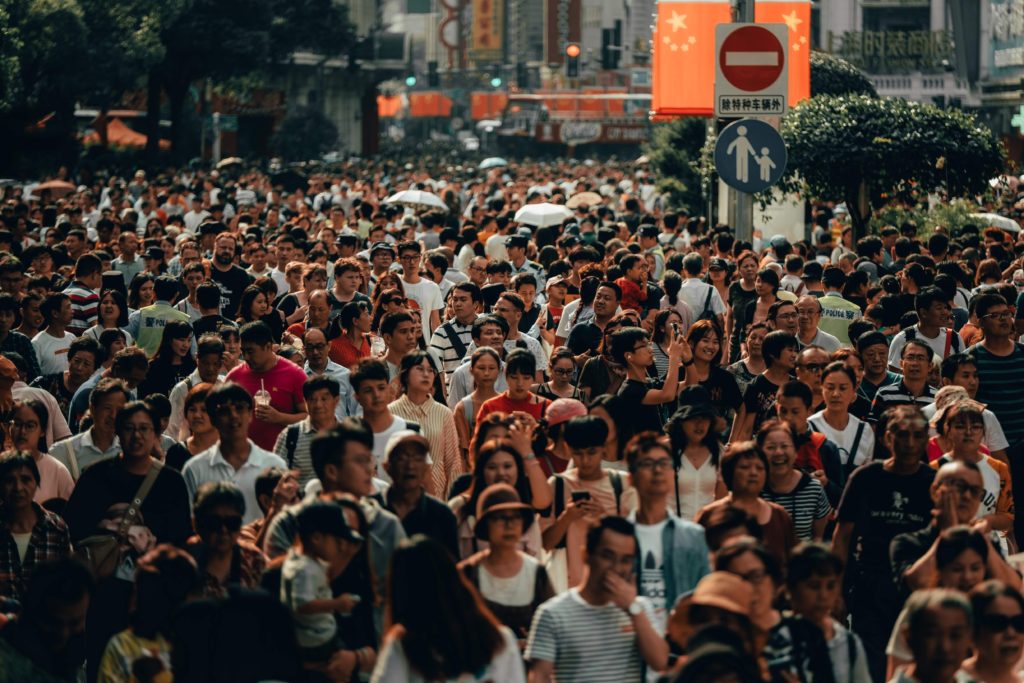
The only way to support such data rate explosion in the world’s cellular networks is to utilize the mmWave frequency bands. In order to solve that, all wireless devices such as smartphones, both high end and low end, and even IoT devices, need in the near future to support communication in the mmWave frequency band. Therefore, mmWave communication, with all their challenges such as shorter coverage and high sensitivity for blocking radio signals by hands or other obstacles, need to be solved by a sustainable and scalable digital beamforming solution optimized from all angles of the system, not only focusing on the radio front end parts, which typically comes into one’s mind talking about mmWave radio communication, but also things such as:
– System architecture including radio and baseband interface
– Digital beamforming algorithms
– Mobility and handover procedures
– Performance and power consumption
BeammWave knows the entire system and delivers the digital beamforming architecture enabling the mass market for mmWave communication in handheld devices opening up the almost endless capacity existing in the mmWave frequency band!