What about the future? What are the new applications? And can we really use this to communicate with exoplanets?
How can operators benefit from digital beamforming? What is the capacity gain and why does it work so much better?
What is mmWave and how do you do beamforming?
The mmWave frequency range is highly suitable for sensing and radar purposes due to the short radio wavelength enabling localization and positioning in the mm to cm range. Joint sensing and communication is being discussed, where a communication system not only communicates but also senses the environment and basically uses mmWave radar for the detection and positioning of objects.
One of the key features of cellular communication is mobility – this allows a mobile terminal to move around between cells while maintaining an uninterrupted connection to the network. Mobility is important in mmWave communication too and particularly so if mmWave communication is to be successful in offloading traffic from increasingly loaded sub 6 GHz carrier frequencies.
Current radio architectures used in mobile terminals for 5G-NR mmWave communication rely on analog beamforming. In analog beamforming the mobile terminal can only transmit or receive in one direction at a time. Therefore, when searching for neighbouring cells, the mobile terminal is allowed to carry out beam sweeping in which it uses up to 8 different beams for detecting signals from every possible direction around it. This translates into the neighbour cell detection time being up to 8 times longer than if the mobile terminal had been capable of receiving from multiple directions at the same time. The same principle applies to signal strength measurements on beams in the serving cell i.e. the mobile terminal is granted additional time for carrying out beam sweeping. Analog beamforming thus results in a less responsive system, where handover or beam change may take a longer time than desired and therefore may lead to reduced end-user experience (end-user throughput, latency, power consumption) and overall system performance (system throughput, capacity). Additionally, it may lead to the mobile terminal dropping the mmWave connection and falling back to sub 6 GHz where capacity is scarce.
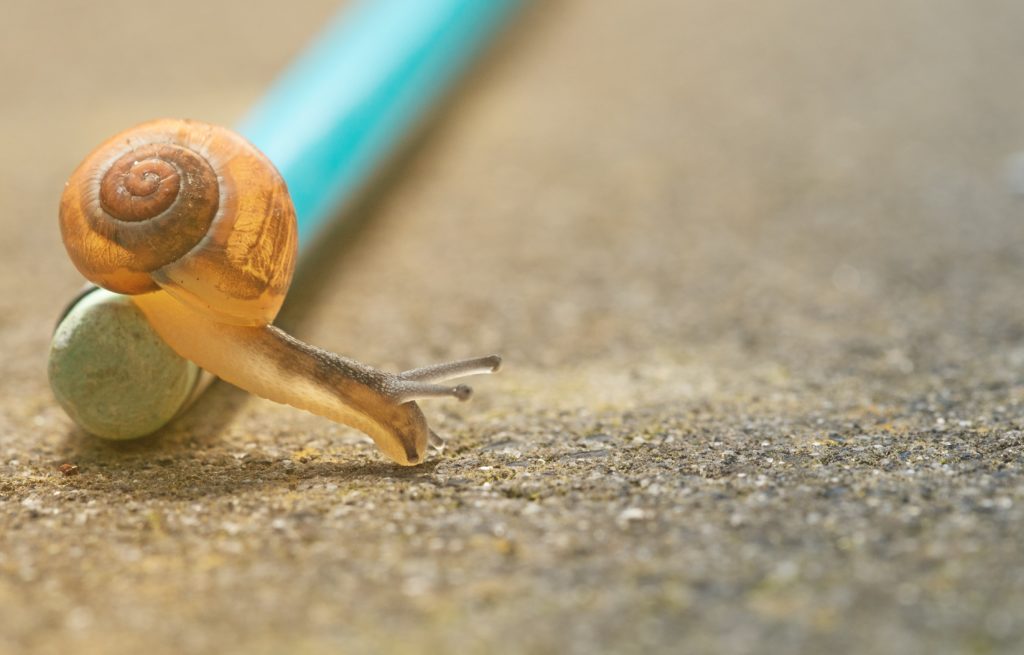
In contrast, digital beamforming allows the mobile terminal to receive from any direction without first having to conduct beam sweeping, and further, to receive from more than one direction at a time, if needed. Therefore, digital beamforming results in a far more responsive system than when analog beamforming is used, leading to better end-user experience and stronger overall system performance by the mobile terminal being connected to the best cell and the best beam at any given time.
BeammWave’s mobility algorithms utilise the above mentioned beam scanning and beam tracking advantages, along with a digital beamforming architecture, to thereby enable a robust mobility operation for mmWave communication.
5G, as well as previous generations of cellular communication, relies on standardized communication protocols for the interactions between mobile terminal and the base station. With new and evolved features, and support of higher data rates, the complexity of the standards has increased for each new generation of mobile communication.
Previous generations of cellular communication standards are using frequencies up to about 3 GHz, for which reliable communication can be maintained using one or a few omnidirectional antennas on the terminal side. With the introduction of NR frequency range 2 (24-71 GHz) (FR2), also known as mmWave, this is about to change as the radio propagation path loss at higher frequencies calls for beamforming based on multiple antenna elements to achieve sufficient sensitivity on both transmitter and receiver sides.
At a first glance it may seem that the requirements for beamforming in NR FR2 would only affect the radio front-end parts of the modem in a handheld device or the radio units in the base station, and by that only the radio frequency requirements of the 3GPP specifications. However, basically all aspects of the communication protocol for NR are affected when introducing mmWave communication.
The figure below shows a block scheme over the cellular modem part in a multi-RAT 5G-NR smartphone, supporting all communication generations from 2G to 5G. The modem can be partitioned into (1) radio components supporting the sub 6 GHz communication (i.e. all legacy communication 2G-4G and also the sub 6 GHz communication mode (FR1) in 5G), (2) radio components supporting the mmWave communication introduced in 5G-NR, and (3) a baseband processor performing all algorithms needed to process a received radio signal to an information signal in the receiver and generate a radio signal from the transmitted information signal in the transmitter. The figure also shows which parts of the radio blocks and baseband processor algorithms that need to be updated for mmWave communication, such as (with reference to respective 3GPP standardization document number)
- Radio frequency requirements (38.101-2)
- Demodulation performance requirements (38.101-4)
- Radio resource management (38.133)
- Physical channels and modulation (38.211)
- Physical layer procedures for control and data (38.213, 38.214)
- Physical layer measurements (38.215)
- Media access control (38.321)
- Radio resource control (38.331)
As can be seen mmWave communication is not only about radio design but it affects basically all algorithms and procedures in the modem, from the physical layer, up to the RRC layer .
Figure: a block scheme of a modem in a handheld device and corresponding parts affected by mmWave communication.
BeammWave understands all these aspects of the standard and how mmWave will impact the system design in handheld and fixed wireless devices, as well as in base stations, and will deliver a sustainable, scalable digital beamforming solution that enables the mass market for mmWave communication for 5G-NR.